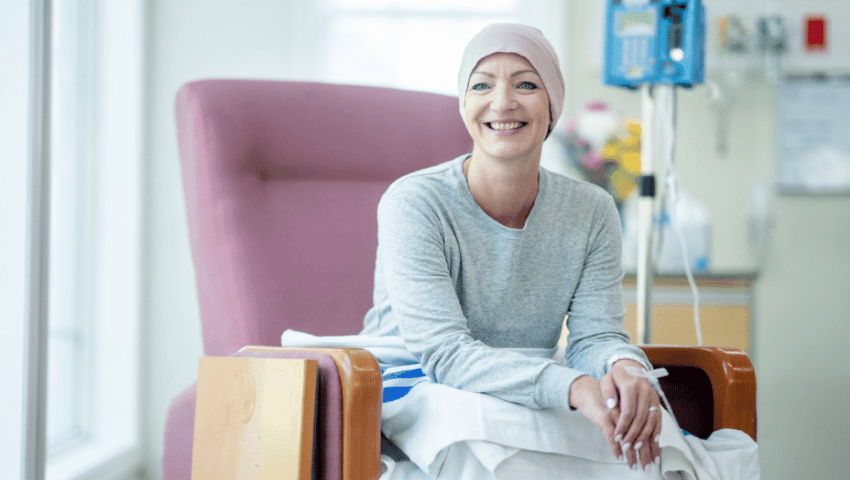
- 05/05/2025
- Dr. Pratik Patil
- 0 Comments
- Blog
Groundbreaking Update in cancer Treatment
Stanford researchers have developed a Molecule that forces the cancer cells to End Themselves. What if cancer cells could be convinced to end themselves—not through harsh chemicals or radiation, but by a signal from within? For decades, cancer treatment has largely relied on attacking tumors from the outside—blasting them with toxic drugs or energy in hopes of outpacing their growth. But cancer is nothing if not adaptable. It mutates, resists, and often returns stronger. In fact, more than 9 million people worldwide still die from cancer each year, despite significant advancements in treatment.
Now, a team of Stanford researchers has taken a radically different approach. Rather than targeting cancer cells with external weapons, they’ve designed a molecule that taps into the cell’s own internal kill switch—essentially instructing the tumor to commit suicide. It’s not science fiction. It’s a lab-tested strategy that could reshape how we think about treating the disease.
The Molecule: How It Works?
At the heart of this development is a lab-made molecule that behaves like a synthetic version of p53, a naturally occurring protein often called the “guardian of the genome.” In healthy cells, p53 plays a key role in regulating cell division and triggering self-destruction when something goes wrong. However, in many cancers, the p53 gene is either mutated or silenced, giving tumors free rein to grow unchecked. The Stanford team, led by Nobel laureate Carolyn Bertozzi, devised a circularized peptide—a specially engineered protein—that can mimic p53’s lethal messaging system, even when the body’s natural version has been compromised.
This synthetic molecule enters cancer cells and delivers instructions that activate ferroptosis, a form of programmed cell death distinct from the more commonly known apoptosis. Unlike apoptosis, which can be evaded by many cancer types, ferroptosis relies on iron and generates a lethal buildup of lipid peroxides within the cell. This process essentially causes cancer cells to rust from the inside out. The precision of the molecule is what sets it apart. Rather than triggering widespread cell death that can harm healthy tissues, the peptide targets the specific vulnerabilities of cancer cells—offering a more refined strategy that may avoid many of the side effects tied to traditional chemotherapy or radiation.
Early lab tests have shown remarkable results. In cell cultures and animal models, the molecule effectively killed cancer cells across multiple tumor types. What’s more, it operated independently of immune signaling or genetic context—meaning it could potentially work across a wide variety of cancers, including those that have previously resisted treatment. The molecule’s structure, being circular rather than linear, also contributes to its stability inside the body, allowing it to remain intact long enough to do its job. Though it’s still early days, the science behind this molecule could shift the treatment landscape by enabling internal collapse rather than external attack.
Why This Matters? Current Gaps in Cancer Treatment:
Cancer’s ability to mutate, hide, and resist treatment has long made it one of the most formidable challenges in medicine. Standard therapies like chemotherapy, radiation, and immunotherapy have saved countless lives, but they are not without significant drawbacks. These treatments often damage healthy cells alongside cancerous ones, leading to a range of side effects from fatigue to organ toxicity. Even when initial responses are strong, cancer cells can develop resistance over time, allowing the disease to return in a more aggressive form.
One of the main culprits behind this resistance is the failure of tumor suppressor systems, especially p53. In more than half of all cancers, p53 is either mutated or turned off. When this happens, cancer cells gain the ability to ignore the usual checks and balances that would normally trigger their death. That means even when patients receive powerful treatments, the cancer can continue to grow because the core biological mechanism for halting it has been disabled. For decades, scientists have tried to find ways to reactivate or mimic p53, but the complexity of the protein and its interactions has made that difficult.
The new molecule developed by Stanford researchers may finally offer a way around this barrier. By mimicking p53’s function without requiring the actual gene to be intact, the molecule sidesteps one of cancer’s most common defenses. Even more promising is its ability to work independently of the immune system, which is a major limitation in some of today’s most advanced therapies like CAR-T and checkpoint inhibitors. This opens the door to reaching cancers that don’t respond to immune-based treatments—such as certain forms of pancreatic, ovarian, or brain cancer—by turning their own biology against them.
The Research Behind It:
The breakthrough came from a collaboration between experts in synthetic chemistry, oncology, and protein engineering. Led by Carolyn Bertozzi—whose previous work on bio-orthogonal chemistry earned her a Nobel Prize—the team focused on designing peptides that are both highly stable and capable of entering cells. They ultimately created a ring-shaped version of a p53 segment, which offers several advantages over its linear counterparts. The circular shape makes the molecule more resistant to degradation, meaning it can survive in the body long enough to reach its target and remain functional once inside.
To ensure the molecule reached only cancer cells, researchers engineered it to bind to a protein found predominantly on the surface of cancerous tissue. Once inside, it triggers ferroptosis by interfering with the cell’s metabolism and prompting an iron-driven cascade of oxidative damage. The researchers noted that this approach worked across a range of cancer types, including cells from aggressive tumors like glioblastoma and triple-negative breast cancer. These are among the hardest to treat with current therapies, which adds weight to the molecule’s promise.
Preclinical testing has been especially encouraging. In mouse models, tumors shrank significantly after being treated with the molecule, and importantly, there were no observed toxic effects on healthy tissue. Researchers also confirmed that the cancer cells did not appear to develop resistance in the same way they often do with chemotherapy. While more extensive testing is needed to confirm this, the molecule’s mechanism—targeting a fundamental metabolic vulnerability—may make it harder for cancer to evolve a workaround. As Bertozzi’s team continues to refine the design, the next major step is preparing the molecule for human trials.
What’s Next From Lab to Patient:
Despite the strong early results, this therapy is not yet ready for human use. Clinical trials are still at least a couple of years away, as researchers must first complete toxicology studies, optimize delivery systems, and navigate the regulatory pathway. Human biology is far more complex than lab models, and what works in mice does not always translate to success in people. Still, the design of the molecule is considered highly promising, and its mode of action offers advantages that existing drugs cannot match. If the upcoming trials are successful, this molecule could eventually be developed into an injectable or targeted drug therapy.
One of the key questions scientists will need to answer in the next phase of testing is how to deliver the molecule most effectively into the human body. Peptides, while potent, are often broken down by digestive enzymes if taken orally, and they don’t always reach the right tissues if delivered systemically. The Stanford team is currently exploring various delivery methods, including nanoparticle coatings or attaching the molecule to antibodies that seek out cancerous tissue. These innovations could allow the molecule to reach deep-seated tumors without harming surrounding cells, potentially transforming it into a safe and scalable treatment.
At the same time, researchers are working to understand the full range of cancers the molecule can target. While the initial studies focused on a broad spectrum of cell types, clinical trials will need to be more specific. That means identifying biomarkers—biological signals—that help predict which patients are most likely to respond. If such markers can be reliably identified, the therapy could be personalized, offering a precision medicine approach that avoids one-size-fits-all solutions. As with any experimental treatment, progress will be careful and deliberate, but the groundwork is strong and the trajectory is hopeful.
The Bigger Picture Rethinking Cancer Treatment:
This research fits into a broader shift in oncology toward smarter, more targeted therapies that cause less collateral damage to the body. For years, the goal has been to develop treatments that not only eliminate cancer but also spare healthy tissue, reduce long-term side effects, and improve patients’ quality of life. Molecules like the one developed at Stanford suggest that the future of cancer care may rely less on external destruction and more on harnessing the internal machinery of cancer cells to turn against themselves. This internal sabotage could represent a quieter, more elegant way to combat disease.
The idea of using a cell’s own survival system as its undoing is not entirely new, but it has rarely been executed with this level of precision. By tapping into ferroptosis—a cell death pathway that cancer cells typically don’t expect—researchers have found a clever route to outsmart the disease. It’s not about overpowering cancer with bigger weapons; it’s about using its own biology to deliver a fatal message. That shift in strategy reflects a growing understanding that cancer isn’t one disease, but many—and it requires approaches that are as adaptable and dynamic as the disease itself.
Beyond cancer, this work also points to new frontiers in synthetic biology and molecular design. If scientists can design proteins that function like natural regulators—yet perform even better—it opens up possibilities for treating not just cancer, but autoimmune diseases, neurodegeneration, and beyond. It’s a glimpse into a future where medicine is not just reactive, but deeply intelligent—where treatments are made with surgical precision and built to work in harmony with the body rather than against it. The Stanford molecule is one step in that direction, and it’s drawing attention for good reason.
A Step Forward, Not a Silver Bullet:
While the headlines are compelling, it’s important to remember that this molecule is still in the research phase. Human trials have not yet begun, and there are many questions left to answer—about safety, delivery, long-term effectiveness, and real-world application. Science is rarely linear, and breakthroughs often unfold in stages, with progress marked as much by setbacks as successes. That said, what makes this discovery stand out is its clear potential and the solid scientific groundwork that supports it. This isn’t speculative theory—it’s a functioning molecule with real effects in living systems.
For patients and families facing cancer, hope often comes with caution. New treatments are always welcome, but only time and rigorous testing can determine whether they truly change the landscape. What’s promising about this molecule is that it doesn’t rely on a miracle—it’s built on a well-understood biological principle, engineered with care, and tested with transparency. It offers a fresh strategy based on internal disruption rather than external attack, and that alone makes it worthy of attention.
If future trials confirm what early studies suggest, this molecule could represent a meaningful step forward in how we treat one of the world’s deadliest diseases. It’s not a silver bullet—but then again, medicine rarely is. What it offers is a smarter approach, rooted in biology, designed to work with the body rather than against it. And that shift—toward intelligence, precision, and internal repair—may be exactly what the next generation of cancer treatment needs
Dr. Pratik Patil stays updated with the latest cancer research to give his patients the best and most advanced care. He believes in treating each patient with personal attention and modern, world-class methods. He also specializes in advanced treatments like targeted therapy and immunotherapy to improve patient outcomes.